High performance, functional materials are a cornerstone for advanced manufacturing and innovative technologies. In particular, the Houston area is home to many chemical manufacturers who use catalytic materials to intensify their processes by making them more efficient, selective and less resource consuming. Catalysts are also used to treat vehicle exhaust, treat waste water and enable renewable energy technologies. The electrification of transportation, and in the long term, possibly the chemical industry, may also require innovative and safe electrochemical storage solutions. Finally, existing catalysts may be pushed to even higher performance by imposing dynamically changing reaction conditions, which is particularly interesting for decentralized, modular reactors.
Grabow group introduction video
Our group integrates electronic structure simulations, transient kinetic models and machine learning approaches to develop computational workflows that help in discovering and designing novel catalysts with tailored properties or enhance catalytic performance by imposing dynamic operating conditions. We also conduct transient kinetic experiments in a Temporal Analysis of Products (TAP) reactor to validate model predictions and to develop dynamic processes . Designing novel materials in silico is an exciting avenue that aligns well with the multi-agency Materials Genome Initiative and current interests to leverage artificial intelligence for data-driven material discoveries that avoid costly trial-and-error experimentation. A recent example of a computational catalyst discovery is a new cost-effective metal alloy catalyst designed for diesel exhaust treatment, for which laboratory experiments show high CO oxidation activity below 150°C and no inhibition by other pollutants.
The necessary computational resources for our research is provided by our 76-node/1,824 core cluster Juniper using Intel Xeon Silver 4116 Skylake generation CPUs. Our group also benefits from shared computing facilities at UH, maintained and managed by the Research Computing and Data Core (RCDC) under the HP Enterprise – Data Science Institute (HPE-DSI). The newest system, Carya, has 9,984 Intel Xeon G6252 CPU cores and 327,680 Nvidia V100 GPU cores integrated within 188 compute and 20 GPU nodes. Carya has 1.5 PB of shared hard-disk based storage and 122 TB of shared flash storage space. RCDC also provides access to Sabine (169 compute nodes with 5,704 CPU cores and 12 GPU nodes) and Opuntia (80 compute nodes with 1,860 CPU cores, 4 GPU nodes, and 3 large memory nodes.
Our Group is also an active member in the following centers:
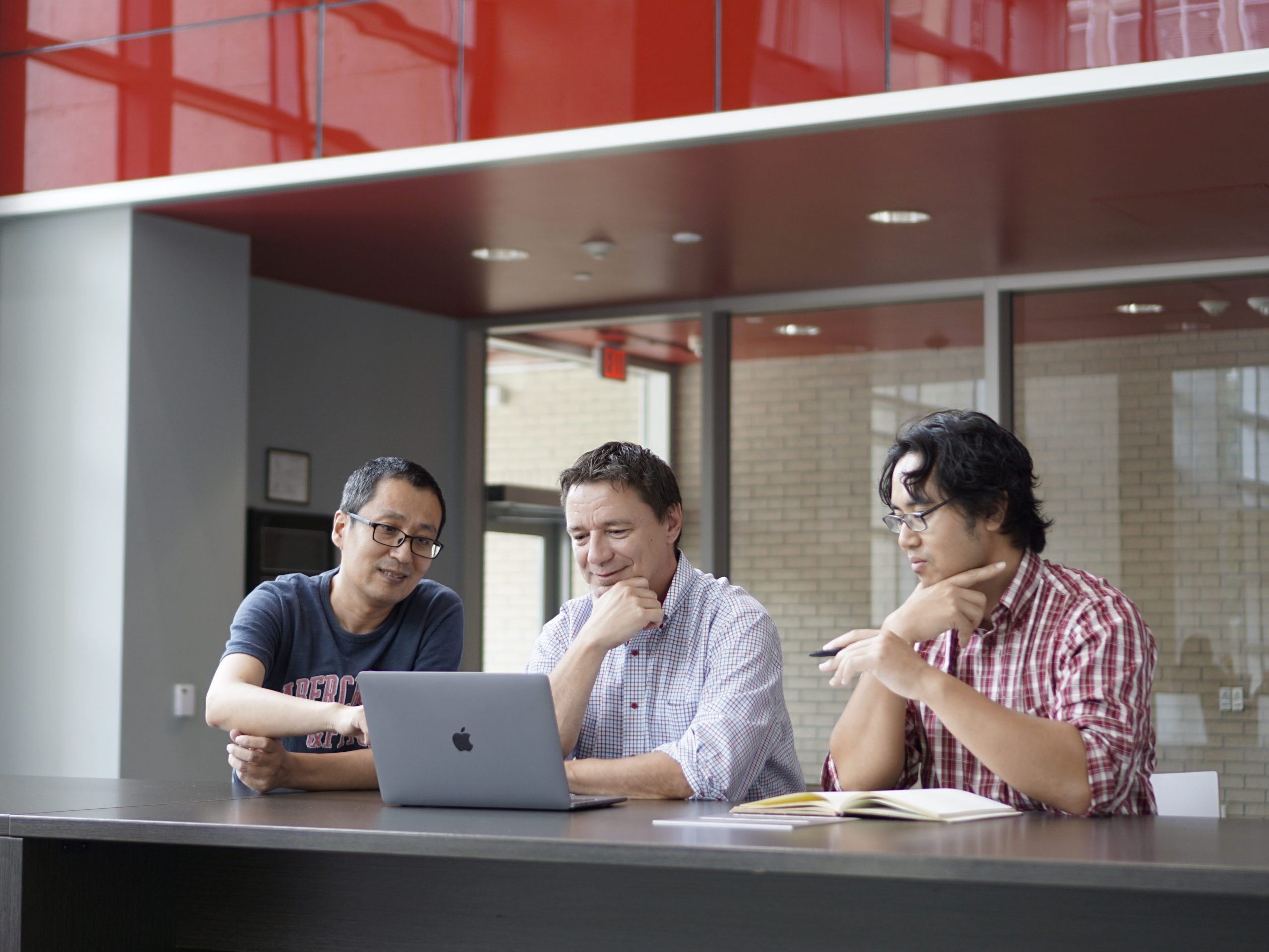